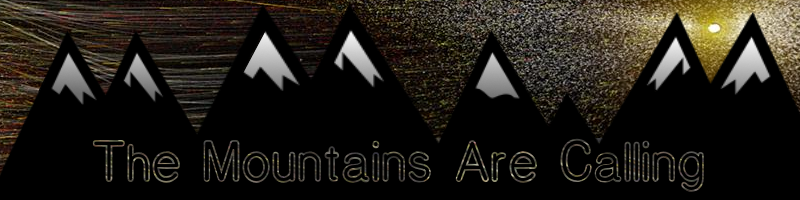
Sunlight & Solar Wind
Stars are the gnarly dynamos of the largely empty universe. Packed with nuclear reactors at their cores, these bodies make life possible.
The number of factors that allow Earth to teem with life is high enough to make one believe in serendipity. We owe everything to our star – the Sun – and our place in its system.
Our planet resides within the habitable zone, famously known as the Goldilocks zone. This band is the distance from a star in which a planetary surface can support liquid water. Too close to the radiant energy of a star and water would evaporate – sorry, Mercury and Venus. Too far away and water would freeze – looking at you, Uranus, and your gas-giant buddies. Earth doesn’t eat the boiling soup or the cold soup but takes the bowl that is just right.
The Sun’s radiant energy arrives at our planet thanks to the light it emits. The process that begins with nuclear fusion and ends with light is long (literally!) and complicated, but it gifts us everything we need to function in the universe. Photosynthesis, the procedure by which plants use sunlight to turn carbon and water into food, fills our atmosphere with oxygen, allowing humans and other critters to survive. Our star’s heat is just right enough to drive the water cycle, which entitles ecosystems across the planet to thrive. This heating force in the right spot isn’t enough on its own, however. If the Earth did not rotate, one side would bake, while the other froze. Life might be able to survive in narrow bands in such a scenario, but things would be dicey.
Further, though the Sun giveth, it can also taketh away. The Earth’s strong magnetic field aids in the long-term retention of our atmosphere. The atmosphere of Mars, conversely, with its weak magnetic field, slowly eroded thanks to a non-light emission from the Sun. Once again displaying the duality of the star’s power, this emission also shields planets in the solar system from deadly cosmic rays (more on this topic later).
If any one of these factors did not exist or slightly altered, you might not be alive to read this article. It’s a remarkable set of circumstances. To celebrate our cosmic good fortune, let’s explore the two emissions most vital for life on Earth: sunlight and solar wind.

Visible light is part of the electromagnetic radiation spectrum. The radiation can differ in frequency and wavelength, which leads to different properties. The lowest frequencies on the spectrum are radio waves, followed by microwaves, infrared, and visible light. As frequency increases, visible light yields to ultraviolet, then X-rays, and the spectrum is topped by gamma rays. Human eyes evolved with the ability to see radiation in the visible-light section of the spectrum, though other creatures can view light in different zones. Although radiation functions as a wave, the mysterious entity also behaves as a particle! Though massless, the quantum of electromagnetic radiation is called a photon. The photon carries the electromagnetic force and is responsible for imparting every bit of light you perceive.
Most of the electromagnetic radiation released by the Sun escapes in the form of visible light, though it also emits photons from other parts of the spectrum. Thankfully, for us, the majority of the radiation comes in the form of visible light, ultraviolet, and infrared. If our star beamed gamma rays at us, we wouldn’t be having this written conversation.
But how and why does the Sun spew visible light?

The journey of a Sun’s photon is a veritable odyssey, one on incomprehensible and seemingly paradoxical time scales.
Photons emerge from the Sun’s core as a direct result of nuclear fusion. At the core, conditions are incredibly nasty, with temperatures of 15 million degrees Celsius and a density off the charts. The Sun is composed mainly of hydrogen. The core is so hot and dense that the hydrogen’s kinetic energy overcomes its natural electrostatic repulsion. When the hydrogen nuclei collide, they fuse, creating helium atoms. One by-product of nuclear fusion in the core is the release of energy. This process results in high-energy photons that will one day light the Earth.
Visible light famously requires just about eight minutes to reach us from the Sun. These massless particles travel nearly 94 million miles in just 500 seconds. Light speed is lightning quick!
But the photon you see on Earth might be vastly older than eight minutes. It might be so ancient that it was created in the Sun’s furnace before humans developed the skill of wearing clothing.
Nuclear fusion in the Sun’s core produces bad photons for Earth: gamma rays. Fortunately, the Sun’s size and density don’t allow the photons to remain in this high-energy state indefinitely. As a photon emerges from the core, it smashes into the next layer of the sun, called the radiative zone. Photons bounce into particles in this zone and then re-emit in a random direction. The photon dances around this massive region, pinging into particle after particle. Each time an interaction occurs, a tiny bit of energy seeps from the photon to the resident particles. Over time, the radiation slowly works its way outward, moving from the radiative zone to the convection zone, before reaching the visible surface of the star, which we call the photosphere. By the time a photon contacts the surface, it has usually lost enough energy to fit into the visible-light section of the spectrum.
The Sun is so dense that scientists estimate a photon can waltz around the inner sections for as long as 170,000 years! When they reach space, they are off to the races, zooming toward us at the speed of, well, themselves. Eight minutes later, we see that ancient photon as visible light.

Visible light and other forms of electromagnetic radiation are not the only particles the Sun belches.
Today’s other headliner might not be quite as vital to life as light, but it certainly plays a significant role in the ability for life to thrive in our solar system. It can also provide quite the celestial light show.
On Earth, the temperature increases layer by layer, as one approaches the core. From the surface of the Sun – the photosphere – to the core, our star mimics this attribute. However, something bizarre and currently unexplainable happens on the Sun. In the image above, you can see scientists label a couple of other layers above the “surface” of the star. Above the photosphere is the chromosphere, which is then topped by the corona. Note the temperatures of these layers. The chromosphere begins to heat to points beyond the photosphere, but things really get toasty as one reaches the corona. The outer portion of the Sun can reach 2 million degrees Celsius! Solar physicists do not currently definitively understand why this phenomenon occurs, but many postulate magnetism plays some role. Even if the cause is not known, one result of this massive temperature spike is well documented: solar wind.
Paricles in the corona become so hot that they reach kinetic speeds greater than escape velocity, meaning the Sun’s gravity can no longer keep them nearby. The particles that manage to exit become part of the ever-blowing solar wind. The streams take the form of plasma that is composed mostly of electrons, protons, and alpha particles. This “wind” moves at a speed of approximately 1 million miles per hour.
One could argue these charged particles are the most important aspect of defining the solar system. The solar wind flows from the Sun in all directions, and it carries with it the Sun’s magnetic field. The concept of a magnetic field being carried by a wind might seem bizarre, but, at its simplest level, one can imagine a magnetic field as an alignment of particles or molecules. The constituents of the solar wind become aligned by the magnetic field of the Sun and then go on a system-wide joyride. The reach of the solar wind – and therefore the magnetic influence of the Sun – is the definition of the size of the solar system, which we call the heliosphere. Where the interstellar medium and the solar wind reach an impasse is known as the heliopause. Inside the heliopause, the Sun reigns; outside is interstellar space. The Voyager spacecraft passed through the heliopause into the unknown in 2012 and 2018, the only artificial objects to exit the solar system.

A constant stream of charged particles from the Sun probably can’t be good for life, right?
The solar wind poses definite threats to technology in orbit around Earth and other places in the solar system. These particles can fry electronics and can pose a threat to the human body. Thankfully, the Earth’s rather robust magnetic field shields our planet from the solar wind. The Moon, on the other hand, features no magnetic field. The solar wind bombards it. Apollo missions tested the surface for the solar wind, finding the soil to be replete with nuclei from the wind.
The solar wind is not all negative, though. In the same way that our magnetic field protects us, the Sun’s magnetic field, via solar-wind propagation, acts as a mammoth safeguard for the entire solar system. Cosmic rays shoot around the universe, far more energetic and deadly than the ones coming from our sun. The Sun’s magnetic field deflects most of these death rays away from potential Earth impacts.


The solar wind is responsible for two other interesting cosmic occurrences dear to the imaginations of space lovers.
Because it is omnipresent throughout the solar system, any body that travels through the heliosphere will constantly encounter the solar wind. Cue Bob Seger’s tune, “Against the Wind.” The prototypical representation of a comet includes a head followed by a tail. As these icy forms pass the sun, they slowly lose portions of their mass, which we see as the tail. If one were to watch a comet over a long period, one would see the tail always pointing away from the Sun. You guessed the culprit: solar wind.
The second effect is a bit more colorful. As we learned in a previous investigation, the solar wind is responsible for auroras! Despite the magnetic field’s ability to deflect most of the solar wind, the nature of Earth’s magnetism attracts what we commonly call the northern and southern lights. At a basic level, one can envision the planet’s field like a giant bar magnet sticking up through and away from the poles. The charged particles of the solar wind can be guided by the “ends” of this giant bar magnet and funneled toward the poles. When the solar wind interacts with molecules in our atmosphere, electrons become excited and begin to glow, forming auroras.
This combination of constant solar wind and the bar-magnet properties of Earth’s magnetic field provides the reason for aurora hotspots in the northern and southern reaches of the globe, hence “northern lights” and “southern lights.” The tourism boards of Scandinavia and Iceland can thank the solar wind for their high rates of glowing skies.

Auroras aren’t always limited to the areas around the poles, though.
Recently, the cosmos gifted a vast range of North America with the northern lights, including areas that typically never experience the phenomenon, such as TMAC HQ in the heart of Ohio.
What caused this deviation from the North Pole? Did the magnetic field shift suddenly? Did the solar wind blip? As we’ll discover in the next iteration of this series on the Sun’s emissions, sometimes our star becomes a bit naughty and things get lit at the afterparty.
Further Reading and Exploration
The Sun – NASA
Our Sun: Facts – NASA
Nuclear fusion in the Sun – University of Calgary
ANCIENT SUNLIGHT – NASA
The solar wind, explained – University of Chicago
Scientists may finally know why the sun’s outer atmosphere is so freakishly hot – Space